Historically, clinicians have relied on extensive personal experience to prescribe training regimens aimed at optimizing patient outcomes. However, a more modern approach emphasizes the use of scientific methods to quantify training load in order to create effective training programs.
Quantification Methods
Various methods have been employed to quantify training load, including:
– Questionnaires
– Training diaries
– Physiological monitoring
– Direct observation
Recently, new indices of training load have emerged, such as the Training Impulse (TRIMP), which incorporates heart rate data alongside training load, and the Session Rating of Perceived Exertion (RPE), which is based on subjective effort ratings and exercise duration. While physiological adaptations to training are well-documented, their direct impact on patient outcomes has not been precisely quantified. Currently, no single physiological marker effectively measures training load or reliably predicts patient outcomes warranting a comprehensive theoretical framework for measuring and monitoring training load.
Theoretical Framework, Training Load, and Individual Variability
Models that aim to quantify the relationship between training load and patient outcomes often conceptualize the patient as a system where training load serves as the input and outcomes as the output. Although this framework is appealing, its accuracy has been suboptimal, likely due to insufficient consideration of individual variability in responses to training. However, this approach could enhance the understanding of how training load influences patient outcomes and improve the effectiveness of prescribed regimens.
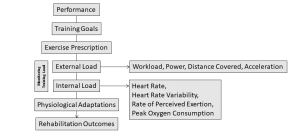
The training load is typically characterized by a combination of intensity, volume, and frequency. These three factors are believed to elicit an adaptive response in the body, which should lead to enhanced performance. To establish a clear causal relationship between the training load and the resulting adaptations, it is crucial to precisely quantify the training load experienced by patients.
There are three distinct types of training loads to consider:
Planned Load: The anticipated training load set before the intervention.
Prescribed Load: The specific training load recommended on a daily basis.
Actual Load: The training load that each patient completes during intervention.
Quantifying Training Load using Physiological Measures
I. Heart rate monitoring relies on the principle that there is a linear relationship between heart rate and steady-state work rate. While absolute measures of intensity are often employed, relative measures may provide more meaningful insights due to the significant inter- and intraindividual variability in responses to different types of exercise.
Using percentage of maximum heart rate is a common method for prescribing exercise intensity. However, Karvonen and Vuorimaa (1988) advocate for the use of percentage of heart rate reserve (equation 1) as a more precise method for quantifying and prescribing intensity. This approach accounts for variations in resting heart rate related to age and fitness level, as well as the fact that maximal heart rate declines with age.
% heart rate reserve = (HR average – HR rest) x 100 / (HR max – HR rest) — equation 1
where HR average is average heart rate measured during the exercise session, HR rest is resting heart rate, and HR max is maximal heart rate.
The day-to-day variation in heart rate is approximately 6 beats per minute, or less than 6.5%. However, controlling for factors that affect heart rate—such as training state, environmental conditions, diurnal changes, exercise duration, hydration status, altitude, and medication—can increase the accuracy of using heart rate as an indicator of exercise intensity.
II. The linear relationship between oxygen consumption (VO₂) and steady-state work rate is well-established, making VO₂ a valid measure of exercise intensity during steady-state exercise. However, this relationship is less applicable during interval or supramaximal exercise bouts. Research by Xu and Rhodes (1999) indicates that when exercise is performed below the lactate threshold, VO₂ increases exponentially until it reaches a steady-state level. In contrast, VO₂ kinetics become more complex when exercising above the lactate threshold, often involving additional physiological responses that can delay the attainment of steady-state VO₂.
To facilitate comparisons of exercise intensities among patients with varying physiological and performance profiles, relative VO₂ (% VO₂max) is frequently utilized instead of absolute VO₂ values. This approach allows for a more standardized assessment of exercise intensity across different individuals. However, it is essential to note that VO₂max is specific to the mode of exercise being performed; therefore, it must be accurately determined for each exercise modality before relative VO₂ values can be effectively used for exercise prescription or quantification.
Research findings suggest that training at intensities above approximately 60% of VO₂max can improve maximal oxygen uptake, with higher intensities yielding similar adaptations in significantly shorter training durations compared to lower intensities. This highlights the importance of tailoring exercise prescriptions to individual capabilities and the specific demands of the exercise mode, ensuring that patients can optimize their training outcomes based on their unique physiological profiles.
Oxygen consumption reserve (VO₂R, equation 2) has been proposed as a more precise method for prescribing exercise intensity compared to % VO₂max.
%VO2R = (VO2 average – VO2 rest) x 100 / (VO2 max – VO2 rest) — equation 2
III. The Rating of Perceived Exertion (RPE) is a subjective scale that allows individuals to assess their exercise intensity based on their own physiological sensations during physical activity. This concept, primarily developed by Gunnar Borg, has been widely utilized in both clinical and athletic settings to help individuals regulate their exercise intensity and monitor their physiological responses.
Validity of RPE in Exercise Contexts
Research has demonstrated that RPE correlates well with various physiological markers during steady-state exercises and high-intensity interval training. For instance, studies have shown strong correlations between reported RPE and average heart rate, as well as acute changes in heart rate during these exercise modalities. However, discrepancies arise in short-duration, high-intensity activities, such as soccer drills and step dance sessions, where the correlation between heart rate and RPE is often weaker.
A meta-analysis conducted by Chen et al. (2002) highlighted that while the Borg scale is a valid tool for measuring exercise intensity, the correlation coefficients between the Borg 6–20 RPE scale and physiological variables are not as robust as previously believed. The weighted mean validity coefficients reported were 0.62 for heart rate, 0.57 for blood lactate, 0.64 for %VO2max, 0.63 for VO2, 0.61 for ventilation, and 0.72 for respiration rate. These findings indicate that while RPE is a useful measure, it may not always align perfectly with physiological responses, particularly in certain exercise contexts.
Understanding the Mechanisms Behind RPE
The variability in RPE responses suggests a need for further investigation into the underlying mechanisms that influence perceived exertion. Research indicates that RPE is not solely a reflection of physical exertion but is also influenced by psychological factors such as motivation, fatigue, and the anticipated duration of exercise. The brain appears to regulate RPE in an anticipatory manner, taking into account an individual’s awareness of their metabolic reserves and the expected endpoint of the exercise. Understanding these factors may enhance the application of RPE in therapeutic settings and improve its reliability as a measure of exercise intensity for patients, particularly those with cardiovascular concerns or other health conditions.
IV. Total Mechanical Work Done (TMWD) is a measure of training load with significant implications, as it is frequently used as a key benchmark in both clinical and research settings. In clinical practice, training is often prescribed and monitored by quantifying its external load, such as TMWD. In research, TMWD is typically used to standardize training interventions that vary in duration and intensity.
A study by Wallace et al. (2014) investigated the relationship between TMWD and total VO2 during a series of diverse training sessions. The findings indicated a strong association between total VO2 and TMWD, leading the authors to conclude that TMWD is a valid measure of training load. This challenges the prevailing belief that internal training load metrics, such as heart rate and perceived exertion, are more effective in representing the training stress experienced by individuals.
However, analyses involving athletes have reported nearly perfect correlations between TMWD and several internal training load metrics, including Training Impulse (TRIMPs) and session-RPE (sRPE). These correlations suggest that any limitations affecting TMWD’s validity as a training load metric may also apply to the internal metrics that are closely associated with it.
Therefore, although TMWD is recognized as a valid measure of training load, its relationship with internal load metrics needs further investigation to fully understand the dynamics of training load and its implications for patient outcomes.
Some researchers emphasize that the manner in which a session is completed may be more important than its TMWD. For instance, instead of maintaining a constant TMWD between sessions, it must be increased as exercise duration increases to ensure the patient’s effort is comparable (Devasahayam et al. 2020). In a controlled study by Nicolò et al. (2014), comparing constant and variable intensity sessions (e.g., using intervals) showed that constant protocols are much less stressful or allow for greater TMWD for the same effort. Additionally, even when TMWD and the interval work-to-rest ratio are kept constant, longer interval durations increase the metabolic strain, or training stress/load.
V. The Banister TRIMP (Training Impulse) is a method for quantifying the physiological stress of an exercise session using heart rate data. The underlying premise is that the elevation of heart rate above resting levels during exercise is proportional to the physical effort expended. To calculate a TRIMP, the following variables are required:
– Training duration
– Maximal heart rate
– Resting heart rate
– Average heart rate during the exercise session
The TRIMP is then calculated using an exponential equation that incorporates these variables (equation 3). The higher the average heart rate relative to the individual’s heart rate reserve (difference between maximal and resting heart rate), the greater the TRIMP value for that session.
TRIMP (w(t)) = duration of training (min) x ΔHR ratio x Y — equation 3
where Y = 0.64e1.92x for males, Y = 0.86e1.67x for females, e = 2.712 and x =ΔHR ratio.
The Y factor is a part of the TRIMP calculation that gives extra weight to high-intensity exercise. It is included to prevent giving disproportionate importance to long-duration, low-intensity exercise compared to short-duration, high-intensity activity. The Y factor is derived from the lactate profiles of trained men and women relative to increases in exercise intensity. The ability to quantify and condense training into a single figure or factor, as enabled by this equation, is particularly appealing for its practical application.
The Banister TRIMP has been widely used in endurance sports to quantify training load and monitor training adaptations. However, some limitations have been identified:
1. The equation uses a general exponential factor based on sex, which may not accurately reflect individual heart rate responses.
2. Incorporating only the mean heart rate for the entire session can underestimate the training load, particularly for interval training where heart rate fluctuates significantly.
To address these limitations, modified TRIMP methods have been proposed, such as:
– Calculating partial TRIMP values for each exercise interval and summing them (TRIMPc)
– Incorporating time spent in different heart rate zones with corresponding weighting factors (Edwards TRIMP)
These modified approaches aim to provide a more accurate representation of the physiological strain experienced during training sessions, especially those involving high-intensity intervals. However, further research is still needed to fully validate and optimize the use of TRIMP methods in quantifying training load and predicting rehabilitation outcomes.
A practical limitation of TRIMP as a measure of training load is its inability to quantify non-aerobic exercises like resistance training. This is due to the disproportionate increase in heart rate during resistance exercise, which does not align with the heart rate responses required for calculating TRIMP. To address this issue, Busso et al. (1990) modified the TRIMP equation by replacing heart rate reserve with %1RM and duration with the number of lifts. Additionally, other approaches to overcome this limitation have included the use of Rating of Perceived Exertion (RPE) to quantify exercise intensity.
VI. Session Rating of Perceived Exertion (sRPE) is a widely used method for assessing training load, proposed by Foster et al. (1996), as an alternative to detailed measurements of heart rate, exercise intensity, and type. The sRPE reflects the overall difficulty of the exercise session and is assessed 30 minutes after the workout. Training load, or session load, is then calculated by multiplying the sRPE by the duration of the aerobic exercise in minutes (equation 4).
Training load = sRPE x duration of aerobic exercise (mins) — equation 4
A study by Borresen and Lambert found strong correlations between different methods of assessing training load, with an r value of 0.76 between TRIMP and session RPE, and an r value of 0.84 between the summated heart rate zone method and the session RPE method. In another study, Impellizzeri et al. reported individual correlations between session RPE and Banister’s TRIMP method ranging from r = 0.50 to r = 0.77. They concluded that session RPE cannot yet fully replace heart rate-based methods for measuring exercise intensity, as it only accounted for about 50% of the variation observed in heart rate.
Although objective physiological measures like heart rate offer a more precise calculation of internal training load, the subjective RPE method still holds value. In situations where heart rate monitors are unavailable or a simpler method for reporting and calculating training load is needed, the RPE approach can provide reasonably accurate assessments of aerobic training load.
Reference:
Mujika I. The alphabet of sport science research starts with Q. Int J Sports Physiol Perform. 2013 Sep;8(5):465-6. PubMed PMID: 24058942.
Borresen J, Lambert MI. The quantification of training load, the training response and the effect on performance. Sports Med. 2009;39(9):779-95. doi: 10.2165/11317780-000000000-00000. Review. PubMed PMID: 19691366.
Passfield L, Murias JM, Sacchetti M, Nicolò A. Validity of the Training-Load Concept. Int J Sports Physiol Perform. 2022 Apr 1;17(4):507-514. doi: 10.1123/ijspp.2021-0536. Epub 2022 Mar 4. PMID: 35247874.
Thurlow F, Huynh M, Townshend A, McLaren SJ, James LP, Taylor JM, Weston M, Weakley J. The Effects of Repeated-Sprint Training on Physical Fitness and Physiological Adaptation in Athletes: A Systematic Review and Meta-Analysis. Sports Med. 2024 Apr;54(4):953-974. doi: 10.1007/s40279-023-01959-1. Epub 2023 Dec 2. PMID: 38041768.
Karvonen, J., & Vuorimaa, T. (1988). Heart rate and exercise intensity during sports activities: practical application. Sports medicine, 5, 303-311.
Xu, F., & Rhodes, E. C. (1999). Oxygen uptake kinetics during exercise. Sports medicine, 27, 313-327.
Alsamir Tibana R, Manuel Frade de Sousa N, Prestes J, da Cunha Nascimento D, Ernesto C, Falk Neto JH, Kennedy MD, Azevedo Voltarelli F. Is Perceived Exertion a Useful Indicator of the Metabolic and Cardiovascular Responses to a Metabolic Conditioning Session of Functional Fitness? Sports (Basel). 2019 Jul 4;7(7):161. doi: 10.3390/sports7070161. PMID: 31277360; PMCID: PMC6681255.
Wallace LK, Slattery KM, Impellizzeri FM, Coutts AJ. Establishing the criterion validity and reliability of common methods for quantifying training load. J Strength Cond Res. 2014 Aug;28(8):2330-7. doi: 10.1519/JSC.0000000000000416. PMID: 24662229.
Renfree A, Casado A, McLaren S. Re-thinking athlete training loads: would you rather have one big rock or lots of little rocks dropped on your foot? Res Sports Med. doi:10.1080/15438627.2021.1906672
Devasahayam AJ, Chaves AR, Lasisi WO, Curtis ME, Wadden KP, Kelly LP, Pretty R, Chen A, Wallack EM, Newell CJ, Williams JB, Kenny H, Downer MB, McCarthy J, Moore CS, Ploughman M. Vigorous cool room treadmill training to improve walking ability in people with multiple sclerosis who use ambulatory assistive devices: a feasibility study. BMC Neurol. 2020 Jan 22;20(1):33. doi: 10.1186/s12883-020-1611-0. PMID: 31969132; PMCID: PMC6975092.
Nicolò A, Bazzucchi I, Haxhi J, Felici F, Sacchetti M. Comparing continuous and intermittent exercise: an “isoeffort” and “isotime” approach. PLoS One. 2014;9(4):e94990. PubMed ID: 24736313 doi:10.1371/journal.pone.0094990
Vermeire KM, Van de Casteele F, Gosseries M, Bourgois JG, Ghijs M, Boone J. The Influence of Different Training Load Quantification Methods on the Fitness-Fatigue Model. Int J Sports Physiol Perform. 2021 Sep 1;16(9):1261-1269. doi: 10.1123/ijspp.2020-0662. Epub 2021 Mar 10. PMID: 33691278.
García-Ramos A, Feriche B, Calderón C, Iglesias X, Barrero A, Chaverri D, Schuller T, Rodríguez FA. Training load quantification in elite swimmers using a modified version of the training impulse method. Eur J Sport Sci. 2015;15(2):85-93. doi: 10.1080/17461391.2014.922621. Epub 2014 Jun 19. PMID: 24942164.
Busso T, Ha¨kkinen K, Pakarinen A, et al. A systems model of training responses and its relationship to hormonal responses in elite weight-lifters. Eur J Appl Physiol 1990; 61 (1): 48-54
Foster C, Daines E, Hector L, et al. Athletic performance in relation to training load. Wis Med J 1996; 95 (6): 370-4